How high do planes fly?
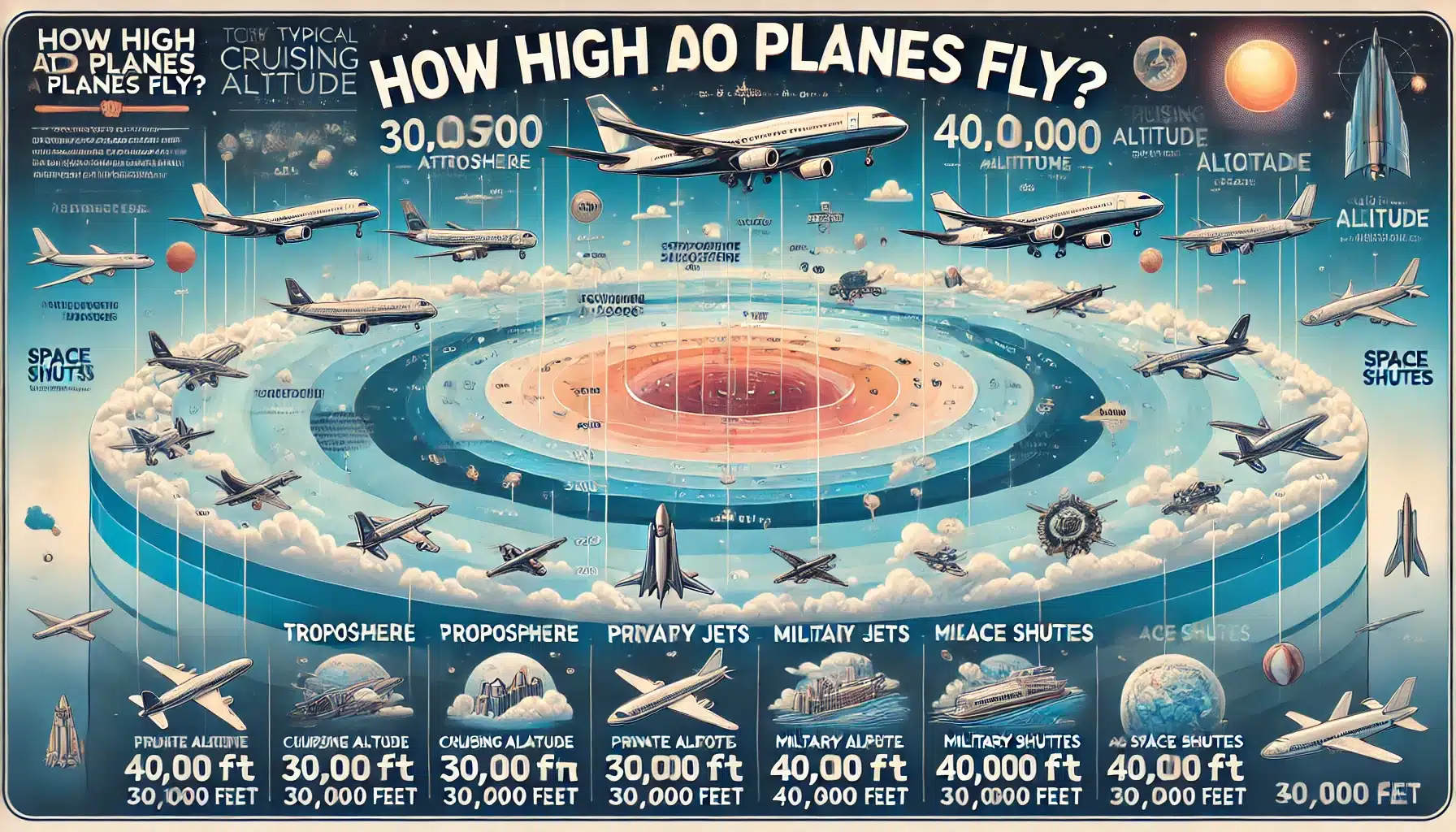
The history of flight and the quest to reach higher altitudes began with the Wright brothers’ first powered flight in 1903, which barely left the ground at a height of about 10 feet. Early aircraft were rudimentary, lacking the power or aerodynamics needed for high-altitude flight. Through World War I and into the 1920s and 1930s, advances in engine technology and aerodynamics allowed planes to climb to a few thousand feet, improving their range and effectiveness. By the late 1930s, aircraft like the Boeing 307 Stratoliner, the first pressurized commercial airplane, could reach altitudes around 20,000 feet, enabling smoother flights above turbulent weather.
World War II spurred a dramatic leap in altitude capabilities as military aviation pushed aircraft to new heights for strategic advantages in combat and reconnaissance. Bombers and fighter planes like the Boeing B-17 and the British Spitfire could operate around 25,000 to 30,000 feet, which offered improved fuel efficiency and the ability to fly above enemy defenses. The pressurization systems developed during the war enabled safer, higher-altitude flight, which became critical for postwar commercial aviation.
The jet age in the 1950s further increased altitudes reached, with commercial jetliners like the Boeing 707 and Douglas DC-8 routinely flying between 30,000 and 35,000 feet. This marked the beginning of regular high-altitude commercial flight, which reduced travel times, improved passenger comfort, and increased fuel efficiency. In the following decades, advancements in aircraft design, materials, and engine technology enabled commercial planes to reach cruising altitudes up to 40,000 feet.
In parallel, experimental and military aviation pushed even higher. In the 1960s, the U-2 spy plane famously reached altitudes over 70,000 feet, designed specifically for high-altitude reconnaissance during the Cold War. The SR-71 Blackbird, another reconnaissance aircraft from the 1960s, could reach altitudes of 85,000 feet, setting records for altitude and speed. The 1970s also saw the introduction of the Concorde, a supersonic passenger jet capable of cruising at around 60,000 feet, where the thinner air allowed it to reach supersonic speeds while maintaining stability.
Today, commercial airplanes like the Boeing 787 and Airbus A350 typically fly at 35,000 to 40,000 feet. Private jets and some military aircraft can reach slightly higher altitudes, up to around 50,000 feet, where there is even less drag. Experimental high-altitude aircraft and specialized drones have pushed the boundaries further, reaching altitudes above 100,000 feet for research and atmospheric studies, while near-space aircraft like the Virgin Galactic SpaceShipTwo can briefly cross the boundary of space at around 328,000 feet.
Each increase in altitude achieved has marked significant technological progress, driven by improvements in aerodynamics, materials, engine power, and pressurization.
Future advancements in aviation may indeed enable travel at even higher altitudes, particularly with the development of high-speed, suborbital, and hypersonic flight technologies. Reaching higher altitudes—beyond the current commercial cruising range of 30,000 to 40,000 feet—would offer several potential benefits. At altitudes above 50,000 feet, the air is thinner, which could reduce drag even further and allow aircraft to achieve faster speeds with less fuel consumption.
This altitude could make hypersonic travel (speeds above Mach 5, or five times the speed of sound) more feasible, shortening travel times dramatically and potentially enabling a flight from New York to Tokyo in just a couple of hours.
One key benefit of flying higher would be the avoidance of nearly all atmospheric weather systems, meaning passengers would experience much smoother flights without turbulence caused by storms or jet streams. This higher cruising level would also place commercial flights above most other air traffic, allowing for safer, more efficient routing and reducing congestion in lower airspaces.
Furthermore, advancements in space tourism and near-space travel could open new opportunities for high-altitude flight. Companies like SpaceX, Blue Origin, and Virgin Galactic are exploring suborbital flights that briefly enter the edge of space, around 328,000 feet, allowing passengers to experience weightlessness and see the curvature of the Earth. For civilians, this could mean a new kind of travel experience, combining rapid transit with the thrilling perspectives of spaceflight.
However, reaching these higher altitudes poses engineering and safety challenges. Aircraft would require highly advanced materials to withstand extreme atmospheric conditions, new propulsion systems for efficiency at high altitudes, and robust pressurization technologies to protect passengers from low-oxygen environments. Environmental impact is another concern, as flying at these extreme heights could potentially affect the upper atmosphere and ozone layer.
While traveling at higher altitudes holds significant promise for the future of aviation, realizing these benefits will require significant technological breakthroughs in materials, propulsion, and environmental management to ensure it is safe, efficient, and sustainable.
There are several potential drawbacks to flying at higher altitudes that would need to be addressed before such travel becomes mainstream. One major concern is the engineering and technological challenge of building aircraft capable of operating safely in the extreme conditions of the upper atmosphere. As altitude increases, the air becomes much thinner, which reduces available lift and requires significantly more advanced, powerful engines and materials that can withstand high speeds and pressures. This leads to increased design complexity, higher production costs, and more expensive maintenance.
Another significant drawback is passenger and crew safety. At extreme altitudes, cabin pressurization requirements become much more stringent, as any failure in pressurization at high altitudes would expose occupants to dangerously low oxygen levels and low temperatures. Rapid decompression at such heights could have severe consequences, making aircraft design and emergency systems far more complex and costly to ensure safety.
Environmental impact is also a concern. High-altitude flights could release emissions into the upper atmosphere, potentially affecting climate and contributing to atmospheric degradation, especially around the ozone layer. Emissions released higher up can remain in the atmosphere for longer, compounding their environmental impact. The industry would need to create sustainable propulsion systems or carbon-offset strategies to mitigate these effects.
Costs are another issue, as high-altitude flights would likely be more expensive to operate, both in terms of fuel efficiency and aircraft design requirements. This could translate into significantly higher ticket prices for passengers, making it an option limited to premium or specialized travel markets rather than accessible to the general public.
Finally, regulatory challenges would arise from increasing air traffic at extreme altitudes. Coordination between aviation authorities and potential space agencies would be essential, as these high-altitude flight paths might overlap with those used for space missions and low-Earth orbit activities, raising concerns about traffic management and safety.
In summary, while high-altitude travel offers exciting possibilities, the drawbacks include engineering challenges, safety concerns, environmental impact, increased costs, and complex regulatory requirements. Addressing these issues would be critical before such flights could become a practical, widely accessible reality.

Staff
Writer